News•December 7, 2014
How Scientists Unraveled the El Nino Mystery
The TAO buoys collect real-time data for improving the detection, understanding and prediction of El Nino. Credit: NOAA
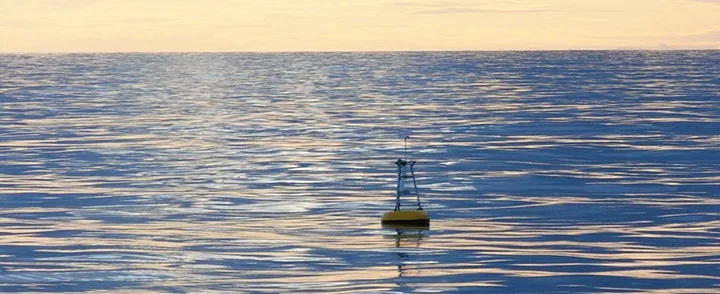
By Daniel A. Gross
It was January 1997, and the Pacific was turning pink. The color was spreading from deep beneath the surface, starting near New Guinea and creeping east toward the coast of Peru — a distance of nearly 11,000 kilometers. On the map, it seemed as though the patch might come to encompass the entire ocean, growing and rising, expanding at the surface almost like the film of an oil spill.
In the actual ocean, there wasn't much to see. David Pierce was looking at a map superimposed with projected ocean temperatures, and pink was a representation of anomalous warmth in the Pacific. The map told Pierce that according to the climate model developed by him and his colleagues at Scripps Institute of Oceanography, huge swaths of the southern Pacific were about to heat up.
A warmer ocean would mean a lot more than better surfing in Baja California. In fact, it would mean torrential rainfall across South America, record winter warmth and wetness in the United States, and $4 billion of damage in the country of Ecuador alone. Climate scientists had even found that through long chains of cause and effect, warm Pacific temperatures were linked to warm air over the Arctic and cold air over Russia — regions that were literally half a world away.
It felt a bit unreal to Pierce that nearly a year in advance, a computer could identify such a drastic development in the climate. The forecast was far from certain knowledge, but if it proved correct, a big El Nino was brewing. Temperature anomalies started in the Pacific, but they could have repercussions across the planet.
Pierce remembers that back in 1997, there weren't many forecasters focused on El Nino, and the few existing climate models were sluggish and tedious. So he wasn't sure that any of his scientific colleagues had yet seen results like this. It gave him an odd and potent feeling, like he had a private glimpse into a likely future. “It was part wonder and part responsibility,” he says. “This thing was coming and it would disrupt lives and economies across the planet, and I realized I might be the only one on Earth who knew it.”
* * *
El Nino is far older than its name, dating back thousands of years according to geological records. Long before climate scientists started to study it, South American fishermen knew how to read it in the water. In a strong El Nino year, the eastern Pacific can rise 30cm and feels palpably warmer to the touch. Species of fish usually caught in June are netted in December. Epic rains fill coastal deserts with lakes, and vegetation sprouts where there should just be dirt.
On the west coast of South America, residents have long interpreted warming water as the sign of an impending El Nino. By the time coastal temperatures rise, however, the phenomenon is typically well on its way. The problem scientists are still trying to solve — the one Pierce was working on in 1997, and that has given researchers special trouble in 2014 — is how to foresee an El Nino many months or years in advance. This is more than a question of pulling out the umbrella before the rain comes. It's a question of understanding a pivotal and fundamental part of the Earth's climate.
Explore this interactive graphic here.
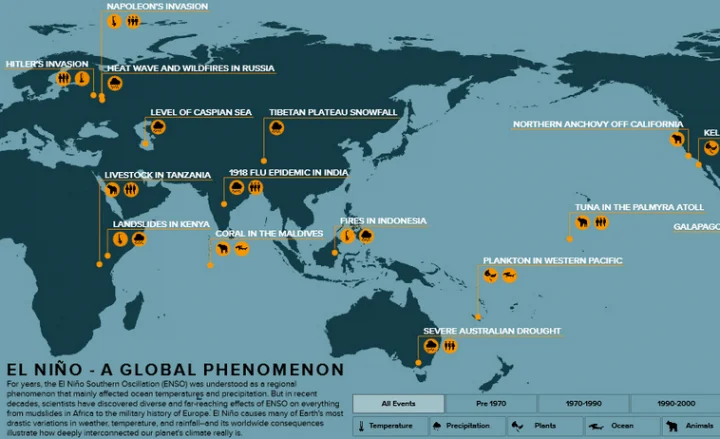
Here's the basic idea of El Nino. Every few years, a relatively warm patch of water — the pink on Pierce's map — forms beneath the Pacific. The difference is just a few degrees, but in a global context, the extra heat can transform the seasonal climate. Some years, the warm region spreads outward and eventually upward, where it meets with easterly trade winds. This creates a feedback loop. Warm water undermines easterly winds that normally enable an upwelling of cool water, which amplifies warming and in turn further slows the winds. It's this moment of atmospheric-oceanic coupling that helps scientists define the start of El Nino.
Wind and water exist in delicate balance with the rest of the climate — so El Nino sets off a cascade of effects. Among its most drastic consequences are increased temperatures along the Pacific and heavy rains along South America's western coast.
That's only the beginning, however. El Nino must have transformed the harvest and thus the fortunes of the Incan Empire. In the 16th century, the Spanish conqueror Pizarro repeatedly attempted to sail down the Peruvian coast, but historical records suggest he failed until El Nino's winds came. It was only after Pizarro's time that fisherman named the yearly rains and warming for the child of Christ, because they tended to arrive around Christmas.
In a tangled and interconnected system like the climate, a seemingly regional phenomenon like El Nino becomes global—and so does the science deployed to understand it. In the 19th century, El Nino was understood as warming in the Pacific, but its secondary effects (if any) were a matter of debate. Scientists were just starting to consider that patterns of regional weather might be connected over vast distances. Increasing air pressure in one part of the world, for instance, was surprisingly correlated with decreasing air pressure elsewhere in the world.
Credit: Climate Prediction Center-NCEP, NOAA
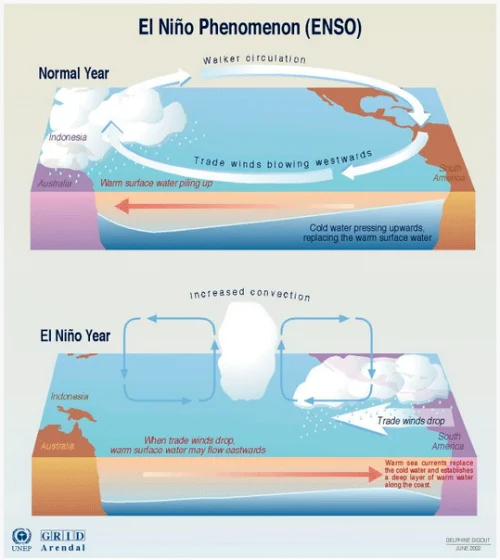
Climate science developed regionally, like pieces of a puzzle that have only gradually been put together. In 1895, Victor Eguiguren postulated a link between heavy rains in Peru and the El Nino cycle. Early in the 20th century, Gilbert Walker theorized a link called the Southern Oscillation between atmospheric pressure in the Indian and Pacific oceans. These pieces were refined independently until the 1960s, when Jacob Bjerknes put them together and found he had a match: The Southern Oscillation and El Nino could be understood as two faces of the same phenomenon, soon known as the El Nino Southern Oscillation (ENSO). Fluctuating pressure provided a causal link between the behavior of wind and water. It also helped explain cooling phases called La Nina, which alternated with El Nino years.
ENSO's impacts have proven to be subtle and far-reaching. The climate historian César Caviedes argued that ENSO helped chill the winter of Napoleon's march to Moscow, just as it helped freeze the soil during Hitler's attempt at the same maneuver. It contributed to drought in the Horn of Africa that, in 1974, led to the collapse of the Ethiopian Empire.
The scope and diversity of these impacts makes it clear that accurate ENSO prediction would revolutionize our understanding of the climate. Advancing science has made it possible to trace El Nino's many consequences in the past and the present, all across the globe. But a crucial question remains: Can scientists reliably see an El Nino coming?
* * *
As 1997 wore on, David Pierce kept his eyes on the news. He and his colleagues at Scripps in California had notified the media that a potentially large El Nino was on its way, and the announcement — echoed by ENSO scientists elsewhere in the world — trickled slowly through the areas likely to be affected.
The Scripps model had started with existing conditions in the Pacific and, using a handful of equations to represent climactic processes, had extrapolated them into the future. In using this method, scientists across the world were departing from the historical standard of ENSO prediction that had dominated in the 1980s. Back then, researchers had looked at past instances of El Nino and tried to guess when the next one would come. This method has its insights, since ENSO is a cyclical process, but it has clear shortcomings: Imagine trying to predict the success of the New York Yankees based on its World Series titles in 1978, 1981, and 1996.
News of the impending El Nino reached Rodney Martinez when he was a scientist in Ecuador, researching climate in a branch of the country's Navy. All the early warning signs for a large El Nino were there, he recalls, from oceanic warming to atmospheric coupling to early shifts in rainfall and temperature. “We had a global consensus in June,” he says, even though most years it takes much longer for climate scientists to fully agree. “Everybody knew that El Nino would be extraordinary.”
El Nino storms cause the 1998 Rio Nido mud slides in northern California, damaging houses and cars. Credit: Dave Gately/FEMA News Photo
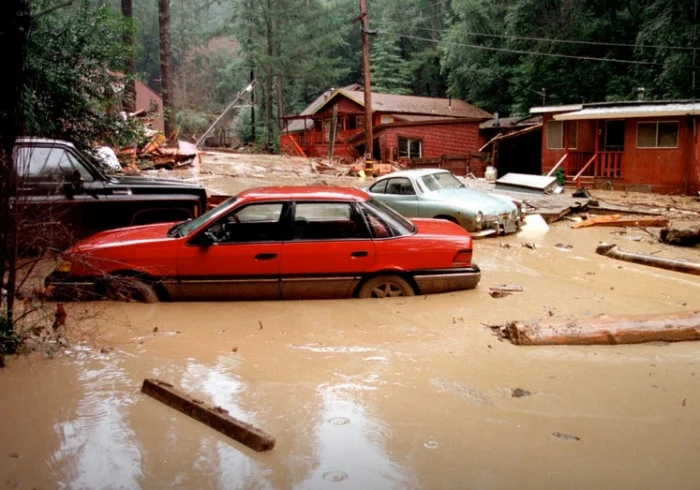
As Pierce saw in the headlines, and as Martinez saw with his own eyes, El Nino struck with a brutal and unrelenting force. On some days, more than 13cm of rain fell on parts of Peru. Streams and rivers rose and widened, and vast pools covered over arid areas where the hard ground was formerly riddled with cracks. Mosquitoes multiplied in standing water; houses and even villages were washed away by flooding. “Roads, infrastructure along the Ecuadorian coast were destroyed,” says Martinez, who conducted a 1998 damage assessment and now works for CIIFEN, a UN-funded El Nino preparedness organization. “There were isolated people, all of the sanitary and water supply infrastructure collapsed.”
Humans have only been keeping climate records for a few decades, so every instance of El Nino teaches scientists a great deal, but the El Nino 1997/98 was especially pivotal. It was the first event of its size that scientists really saw coming. Yet even with the foresight, its effects killed more than 2000 people and caused over $30 billion in damage worldwide. Put simply, El Nino 1997/98 made policymakers pessimistic and scientists optimistic.
Martinez remembers that he and his colleagues in South America struggled to get the word out. “You can have the best models in the world, but that does not necessarily mean that you will reduce the impact in a particular region.” As a result, many national and international programs to prepare for and adapt to El Nino began in 1998.
On the other hand, David Pierce remembers that back then, only a few groups were working on ENSO prediction. Partly thanks to the buzz generated by El Nino 1997/98, research has come a long way. These days, ENSO is a much larger part of academic and national research programs, and there are more than 20 major climate models that help predict it. Many model the entire climate, not just El Nino, and thanks to computing power and advancing research, their complexity make the 1997 Scripps model look rather crude in retrospect. Not every El Nino gives as strong a signal as 1997/98, and newer models aim to extract a prediction even when the warning signs are weak. That was exactly the challenge in 2014.
Residents of Laguna Niguel, California, work together to save the belongings of a condominium that was heavily damaged in a landslide resulting from El Nino storms. Credit: Dave Gately/FEMA News Photo
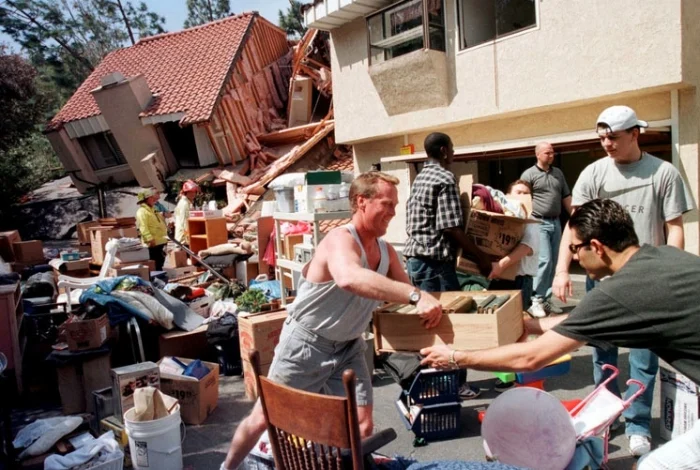
Still, complex models create new challenges: Scientists don't always understand how they work, or how to improve them. Which brings us to the present, when even after two decades of advancing climate science, uncertainty can win the day.
* * *
Early in 2014, the water beneath the Pacific started getting warmer. Data from buoys and satellites, many deployed by the National Oceanic and Atmospheric Administration in the United States, fed into climate models around the world, which produced a fairly uniform result: an El Nino seemed to be brewing, with approximately 75 percent likelihood of developing.
ENSO predictions have come a long way since the 1990s, but there's plenty of work left to do. A 2012 survey of many ENSO models showed that 6-month forecasts have slowly but steadily improved since the 1990s. Even so, thanks to limitations of computer power, data quality, and model reliability, however, the 2014 forecasts were tentative.
In the spring of 2014, word of a potentially developing El Nino reached media organizations. From there, the news spread quickly and loudly. Many publications announced an impending “monster” El Nino, while some scientists went so far as to compare 2014 conditions to the destructive winter of 1997/98.
And then — very little happened. Waters near the coast of South America warmed up, and rainfall spiked in some regions near the coast. But as autumn began, atmospheric coupling still hadn't occurred, leaving many scientists scratching their heads.
A model is a description of the world in miniature, built of a series of equations taken to be fundamental. These equations can't be solved for a specific answer, but they can be tested in simulation and averaged fro a good guess at the future.
The obvious problem is that the climate is layered and complicated. Climate models aim to simplify real-world phenomena, but sometimes, says University of Exeter climate scientist Mat Collins, “they are almost as complex as the real world.”
The very latest 2014 predictions tell us that El Nino still hasn't begun, but warm water anomalies continue in the Pacific. Most forecasters — now more cautiously — still expect El Nino to arrive in the next couple months.
Regardless of the outcome, future models will need to reckon with the bizarre mixed signals that have emerged in 2014. Although the general principles of climate models seem sound, many scientists suspect something crucial is misrepresented or missing. “Every time we have a new El Nino event, we have a revision of the theory about how El Nino seems to be working,” says Collins. These new models may be radically different from their predecessors. “It's usually more than tweaking the old model,” says Anthony Barnston, who co-authored the 2012 paper analyzing various ENSO forecasts. “It's usually different physics.”
Perhaps we don't study climate phenomena despite their complexity, but because of their complexity. The science of El Nino provides some profound insights about the climate at large. ENSO models continue to vex scientists for the very reason that ENSO seems worthy of study: Its impacts can be vast, global, and dizzyingly diverse.
Daniel A. Gross is a writer and public radio producer based in Boston. Greg Laden contributed additional reporting.
This story was first published on www.roadtoparis.info and republished with permission.